In December 2005, Richard Gaitskell flew from Providence to Rome. He rented a compact Italian car that could barely contain his 6’ 3” frame and drove an hour east to the Gran Sasso d’Italia, a nineteen-mile-long mountain chain located in one of the country’s largest national parks. At the base of a mountain there, a guard checked Gaitskell’s identification papers and rolled open a hulking metal door. Gaitskell then drove inside the mountain.
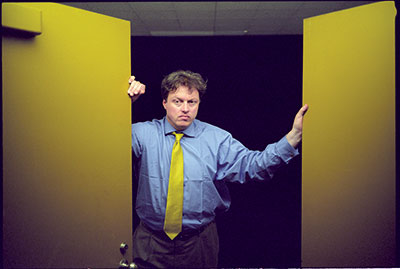
He wound his way through a dimly lit tunnel and pulled into a small parking lot in front of a tube-shaped building. Inside, dozens of scientists wearing lab coats and hard hats scurried about. Above them water dripped from the moss-covered underside of the mountain. It all looked like one of those underground lairs in a James Bond movie, the ones from which bad guys scheme to take over the world.
As a Brown physicist, Gaitskell had traveled into the mountain with a less nefarious plan. Over the next several months, he and a team of researchers from around the world set up a research station aimed at solving one of the greatest mysteries in physics. They cooled fifty gallons of xenon gas to -minus 100 degrees Fahrenheit, turning it into a liquid they then poured into a large steel-and-Teflon cauldron. On the lid of the cauldron were dozens of square, quartz-coated tiles, each one attached via an electrical wire to a computerized sensor. Gaitskell and his colleagues encased the cauldron in an airtight lead chamber with eight-inch-thick walls. When the device was complete, Gaitskell drove back out of the mountain and headed home to Providence. Depending on what data his device gathered over the next year or so, Gaitskell might just make scientific history. He might even win a Nobel Prize.
For decades physicists like Gaitskell have known something the rest of us do not: the universe doesn’t make any sense. Take the stars, for example. They travel around the universe a lot faster than the fundamental laws of physics suggest they should.
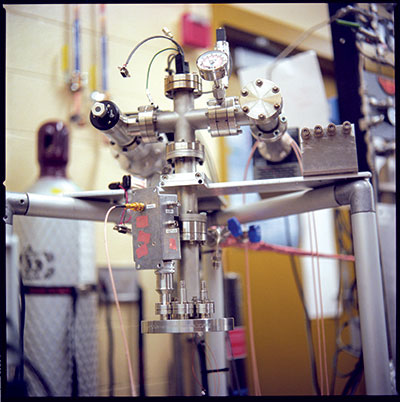
The astrophysicist Fritz Zwicky first noticed this back in 1933 while observing Coma, a cluster of galaxies hundreds of millions of light years away from the earth. Like most such clusters, Coma had a center around which all the individual galaxies rotated. Zwicky observed that the galaxies on the cluster’s outer rim were moving as quickly as those close to its center.
Everything he knew about the physical world told him this should not be. Because most galaxies in a cluster are near its center, that’s where its mass is greatest, and that’s where the gravitational pull is strongest. Because of this concentration of mass and gravity, these central galaxies pull one another around at a relatively rapid speed.
But as you move out from the center of a cluster the number of galaxies and the amount of mass declines. Gravitational pull drops with it, and, in theory, galaxies on the outer margins of a cluster should orbit more slowly. There just aren’t enough nearby galaxies on which to grab a ride.
Zwicky reasoned that something had to be out there that he couldn’t see, some kind of unknown matter with enough mass to bring the gravitational pull on the outer galaxies into balance with the pull in the center of the cluster. This substance came to be known as dark matter.
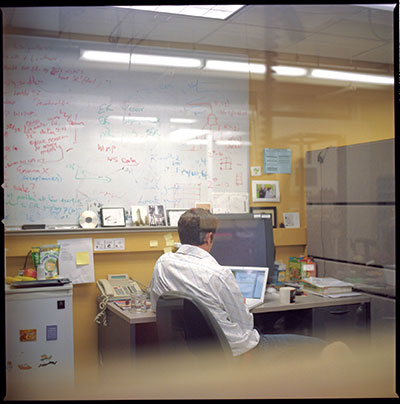
Physicists now theorize that dark matter comprises about a quarter of all the matter in the universe. By contrast, atoms—the stuff that makes up what we see—comprise only 4 percent. (Dark energy, another mysterious substance, makes up the remaining three quarters.) It’s likely, in fact, that two or three dark-matter particles are floating around in your average cup of tea.
Not that you’d know it. No one has ever seen dark matter. It remains invisible to even the most powerful telescopes so its existence can only be inferred. Right now it’s more theory than reality. Most instruments detect atomic matter by sensing the light generated from the charge that’s produced when atoms collide. But dark matter has virtually no charge. When it interacts with atomic particles, no visible light is given off. For the most part, atoms and dark matter go right through one another, like ships passing in the night. Even though dark matter is all around us, even traveling through our bodies, we never know it.
The contraption Gaitskell has built in Italy’s Gran Sasso National Laboratory promises to be the most sensitive detector of dark matter yet. His is one of about a dozen experiments around the world that are currently looking for dark matter. It’s a furious competition with nothing less at stake than fame and glory. Most scientists consider Gaitskell and his team to be underdogs. Gaitskell thinks they’re wrong.
After graduating from Oxford in 1985 with an undergraduate degree in physics, he went to work as an investment banker. His father had been in finance, but the real factor driving Gaitskell’s decision was money. The banks were flush with cash, and, he says, were “offering what seemed like insane amounts to go work there.” He was put on the aerospace and defense-industry desk because, he says, “It was like, ‘Oh, Rick, you know about numbers. You must know about engineering.’”
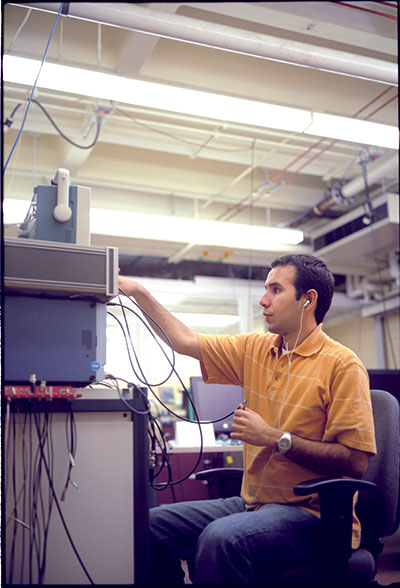
For four years, he lived the good life. He vacationed in Barbados, the Dominican Republic, and on the east coast of Africa. He lived in the posh London neighborhood of South Kensington. Eventually, though, accumulating all those riches lost its appeal. “It felt like it wasn’t a profession any more,” he recalls. “It was more noses in the trough. Greed became boring.” He returned to Oxford, where he began working on dark matter. He now made very little money, but he no longer cared. “I was spending all my time in the lab,” he recalls, “so I really didn’t have anything to spend money on anyway.”
Still, a little of the investment banker remains. In a nod to the go-go 1980s, Gaitskell wears bright red suspenders and colorful ties and socks. But they don’t always match. One day this spring he could be seen in green corduroys, a yellow-and-blue striped shirt, a yellow knit tie, and brilliant red socks. Gaitskell is big and ruddy-faced. He sports a mane of graying black hair that’s usually disheveled, thanks to his habit of running his hand through it regularly.
After getting his advanced degree in particle physics at Oxford, Gaitskell took a post at University of California, Berkeley. When he heard about a new project that was starting up, the Cryogenic Dark Matter Search (CDMS) project, he eagerly signed on.
In 1999 Gaitskell flew from California to Minneapolis and then drove to an abandoned mine site in the northern Minnesota town of Soudan. He donned a yellow hard hat and squeezed with nine other people into an old-fashioned mine elevator that resembled a wooden cage. The elevator descended 2,400 feet into the mine and deposited the scientist in a tunnel where miners once bored for iron. He walked over to a giant door, which swung open to reveal a large cavern blasted from the side of the tunnel. It was crammed with scientific equipment and physicists.
This was the Soudan Underground Laboratory, the site where Gaitskell and the rest of the CDMS team believed they had the best chance at detecting dark matter. The surface of the earth is constantly bombarded with cosmic rays, radiation left over from the Big Bang. When cosmic rays hit the earth, they kick up a slew of subatomic particles, everything from gamma rays to electrons, producing “noise” that makes detecting dark matter impossible. Fortunately, one of the best filters of this subatomic cacophony is the earth’s crust. At the CDMS site, the walls of the deep mine reduce the total number of cosmic rays in the environment from trillions to a few million.
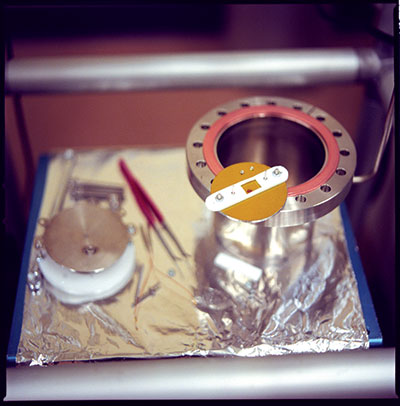
Like most physicists, Gaitskell believes that the key to finding dark matter is finding particles called WIMPs, or weakly interactive massive particles. WIMPs, the theory goes, are the building blocks of dark matter. Physicists estimate that one WIMP has a mass about 100 times greater than a proton, but, unlike a proton, it has no charge, making it virtually undetectable.
Virtually, but not completely. When a WIMP does happen to bump into an atom, it’s thought that the atom’s nucleus will vibrate very slightly. In theory, if the “background noise” is muffled enough, you could “hear” these vibrations.
The CDMS team used germanium and silicon atoms in their experiment. Thanks to their crystalline structure, these elements will generate heat from the vibrations a WIMP triggers. The CDMS researchers took about thirty hockey-puck-shaped slabs of germanium and silicon and piled them on top of one another to create five towers. Each tower went into its own cryogenic chamber, where it could be cooled to a very chilly minus 459.67 degrees Fahrenheit. This essentially froze the germanium and silicon atoms in place, making any vibration generated by a collision with a WIMP much more detectable. Metal caps on the top of each tower work as thermometers, constantly scanning for any discharge of heat. At the time, this was the most sensitive test ever designed to detect dark matter.
But after several years not a single WIMP-atom collision had been measured. Physicists, however, did not expect to get lucky so quickly. Because WIMPs have no charge to draw them to the germanium and silicon atoms in the first place, any collision would have to happen when they accidentally bump into each other. And because WIMPs travel at the glacial pace—in cosmic terms—of one-thousandth the speed of light, they don’t tend to run into other atoms very often. Mathematical models suggest it could take as long as 100 years for one WIMP to bump into a kilogram of germanium or silicon.
To improve their odds physicists would like to stash more germanium and silicon crystals into more noise-free locations. The entire approach is similar to buying lottery tickets—the more you have, the more likely you are to win. But you can also go broke this way. Germanium and silicon are expensive, and when Gaitskell crunched the numbers, he found that the cost of scaling up adequately would be hundreds of millions of dollars. He realized he had to find a less expensive material.
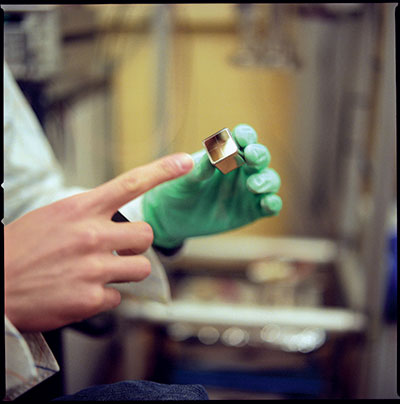
The answer seemed to be xenon gas. Xenon, number fifty-four on the periodic table, is an odorless and colorless gas that is also a very poor conductor of atoms. Because of this, cosmic radiation would not be drawn to xenon, reducing the amount of background noise from the get-go. What’s more, the nucleus of a xenon atom would react much like the nucleus of germanium or silicon: a WIMP would trigger a vibration. And best of all, xenon is relatively cheap at about a $500 a pound. The costs would stay in the tens of thousands of dollars.
While remaining a member of the CDMS project, Gaitskell developed a new experiment using xenon. Researchers from Columbia, the University of Florida, the Lawrence Livermore National Laboratory, Princeton, Case Western Reserve, Rice, and Yale joined him. The site in Italy was selected.
One day in March, Gaitskell and his two graduate students, Luiz de Viveiros and Peter Sorensen ’00 ScM gather in Gaitskell’s cluttered Barus and Holley basement office to analyze the first set of data from the Italian site. The office smells of coffee grounds and sweat: the team has been working twelve-hour days for several weeks.
For the last few months computer sensors in Italy have been recording every interaction the xenon atoms have had with other particles. The data have remained in a locked box until today, but now it’s time for their computers to crunch the numbers. Soon they will know whether they’ve finally been able to detect the presence of WIMPs.
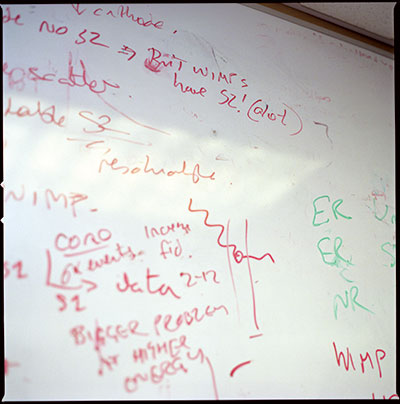
An hour into the process, Gaitskell decides to grab some dinner. He figures the results won’t be ready for some time, and he’s starving. The analysis advances more quickly than he’d thought, however, and while he’s away de Viveiros’s computer finishes its task. Sorensen and de Viveiros stare at the screen, then suddenly begin to cheer and exchange high-fives.
Gaitskell returns during the cheering, disappointed at not having been there at the moment of reckoning. “C’est la vie,” he says, and turns to the graphs Sorensen and de Viveiros have generated. He reviews the data several times before convincing himself that, yes, they have made a major discovery. A broad smile forms across his face.
What the physicists have found is nothing. Absolutely no evidence whatsoever of WIMPS or dark matter. As Gaitskell notes, “It’s been the same for the past seventeen years of my career. We open the box and get nothing.”
But there is nothing and then there is nothing, and in this case, being certain you have found nothing is a big deal. As in past experiments, some cosmic radiation has inevitably managed to infiltrate the cauldron of xenon. In the past, this has made it difficult to differentiate the “noise” given off by a WIMP-xenon collision and that given off by a xenon collision with cosmic radiation.
This time, however, the detector has been able to discriminate between that atomic noise and the presence of WIMPs. The team will do more tests, which will demonstrate that the Italian setup is at least six times more sensitive than any other—six times more effective at ruling out false interactions. “We’re the best in the world,” Gaitskell says. “We’re seeing results no one has ever seen before.” Within weeks, he is making plans to scale up the experiment, hoping eventually to use one ton of xenon.
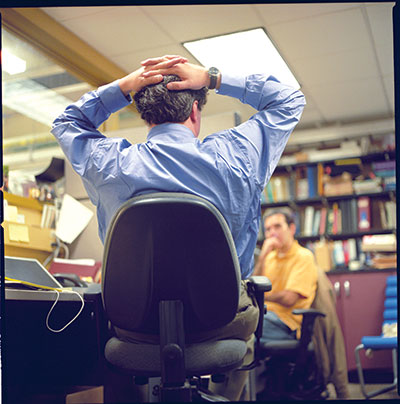
But this finding of nothing raises a vexing theoretical issue. “It’s always possible to build a more sensitive detector,” Gaitskell says, but devising a better mousetrap for finding nothing proves what, exactly? At what point would physicists abandon their hypothesis that WIMPs even exist? The danger is that researchers like Gaitskell spend the rest of their careers thinking they’re chasing a great scientific breakthrough, when in reality they’re just digging themselves deeper into a rabbit hole. “You may get a situation,” he says, “where in 2050 you open up a textbook and WIMPs are mentioned in a footnote that says they were just a fleetingly fashionable theory in the late twentieth and early twenty-first centuries.”
But on this day in March, such nagging questions have no place in Gaitskell’s office. “There’s still been no direct detection of dark matter,” he says. “But we know something today that we didn’t know yesterday.
“That’s science.”
Lawrence Goodman is the BAM’s senior writer.