As one of last fall’s Nobel Prize winners, Craig Mello ’82 has received a level of wealth, publicity, and acclaim seldom granted to a scientist who spends his days observing a microscopic worm. But one day last March, this now world-famous scientist decided to go back to high school. Standing before a student assembly at the public high school in Shewsbury, Massachusetts, where he lives, Mello raised a question he is often asked: how do you win a Nobel Prize?
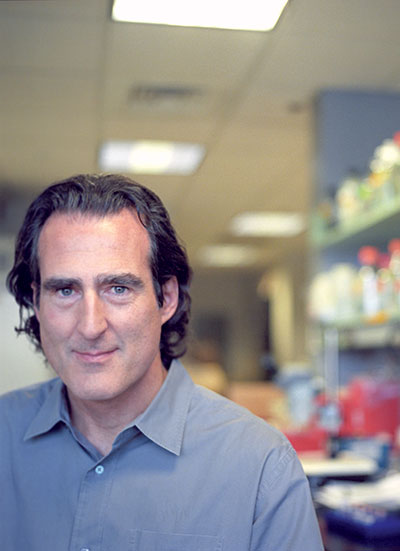
Talk to people and share your ideas, Mello told them. Find something you really love doing and chances are you’ll be good at it. Be persistent, and don’t give up easily.
He then showed the students what a Nobel Prize in medicine looks like. Projecting a picture of his medal onto a huge screen behind him, he joked: “It’s just a big hunk of gold that you keep in a case. I was a little disappointed. I thought I could wear it.” Then he changed the slide. “The other part is this diploma, which is very nice. But it’s in Swedish, so it’s kind of hard to read.”
Modesty aside, Mello and his collaborator, Andrew Fire, have discovered a powerful tool that promises to speed up the development of cures for a host of diseases. By giving medical researchers a way of shutting off genes selectively, they have launched genetics into a new orbit and increased hope for people battling such maladies as HIV and cancer. But Mello, a Howard Hughes Medical Institute investigator and a professor of molecular medicine at the University of Massachusetts Medical School, is an unlikely superstar. He’s the kind of person who finds time between fund-raising in Florida and lecturing in China to judge a local high school science fair, as he also did this spring. At six-foot-three, he had to hunch down when he talked to students about their exhibits, all the better to look them in the eye.
Mello donated some of the $1.4 million Nobel money he and Fire shared to help the town. Specifically, he helped cover the cost of a local ballot measure aimed at bailing out the chronically underfunded Shrewsbury public schools, which his four children attend. And he wants to use his fifteen minutes of fame to add his voice to those warning of impending environmental and social crises and to support increased funding for scientific research. “Innovation is really our hope for the future,” he says. And he adds, “I think the most important thing we can do is educate our kids.”
Caenorhabditis elegans is a common roundworm about a millimeter long. Five Nobel Prizes have relied on this animal. Researchers love it because C. elegans is simple—a mere 1,000 cells. It is transparent, which means its cell division is easy to see. It has a nervous system and muscles just as humans do, but in a stripped-down form. Scientists have long known that if they can understand the biological mechanisms of this worm, they are well on their way to understanding similar systems in more complex animals such as humans. In fact, researchers are so enamored of C. elegans that an entire community of biologists calls itself “worm people.”
Frank Rothman, an emeritus professor of biology as well as former Brown provost, is a worm guy. He was also one of Mello’s teachers at Brown. One day in the mid-1990s, Rothman decided to look up his former student to talk worms. Rothman had just stepped down as provost and was eager to plunge back into his research, which involved C. elegans. As he reviewed the latest scientific papers, he kept coming across Mello’s name. Mello, he recalled, had taken two of his courses: an introduction to molecular and cell biology and an advanced graduate course in biochemical genetics. Rothman had been impressed enough by Mello’s work in the introductory course that he asked him to become a teaching assistant for the same course the following year. “He was always extremely interested and inquisitive,” Rothman recalls. “Very determined to learn as much as he could. He would ask questions, and if he didn’t like the answers, he’d keep after you.”
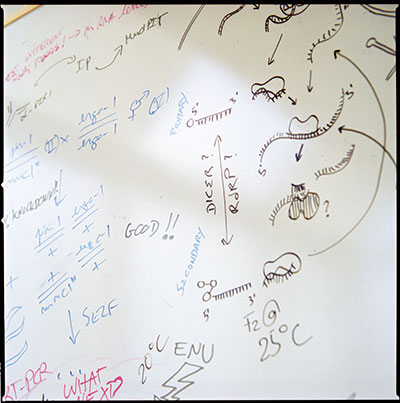
Back in the lab after his stint as the University’s top academic administrator, Rothman decided to catch up with Mello. “I needed worms,” he says, “and a special type of Petri dishes. I could have written for the worms and bought the Petri dishes, but I thought it would be nice to see Craig again.” During Rothman’s visit, the two scientists talked about Mello’s work, which focused on studying cell development in C. elegans. He was particularly interested in why and how embryo cells differentiate. A single-celled embryo contains all the genetic information needed to create a complete organism. When it divides, some of the new cells become specialized, forming nerve cells, for example, or muscle cells, or intestinal cells. Mello wanted to know how this happens, and his experiments involved deactivating the specific genes that were generally believed to govern the process of differentiation.
“One good way to see what a gene does,” Rothman explains, “is to turn it off and see what doesn’t happen.” Just as you can identify the function of a fuse in your house by turning it off and watching which lights go out, scientists find out what a gene does by deactivating it and watching the organism to see which traits disappear.
To understand what happened next, you must know a bit of molecular biology. Think of a cell’s DNA as a master blueprint; its molecular structure encodes the information that drives all of an organism’s biological processes. Discrete linear sequences of DNA—what we know as genes—code for particular characteristics. If you think of DNA as plans for an entire house, an individual gene might specify a small detail of one room. But as in architecture, the blueprints don’t do the actual building; workers do. To get the plans to the workers, cells rely on messenger RNAs, or mRNAs. These encode the information from the DNA and deliver it to the builders, which are complexes of RNA and proteins called ribosomes. Using the genetic information encoded in mRNA, ribosomes link amino acids together in a specific order to form the proteins making up an organism.
Mello’s research was based on intercepting messengers carrying a particular genetic code, preventing them from delivering their piece of the blueprint. He did this by using a tool called anti-sense. Anti-sense is made up of single-stranded RNA molecules with chemical sequences that precisely complement the targeted mRNAs (which are called sense). When scientists inject this anti-sense into cells, it binds chemically with the targeted mRNAs and deactivates them. Doing so shuts down the activity of that gene.
Mello explained his experiments to his former teacher and then described a surprising phenomenon he was observing that could not be explained by any conventional way of thinking. As Rothman recalls, the genes Mello turned off remained off for a long time, even into the next generation of worms. Mello wondered how this could be, as he knew that the agent he was using to turn off the genes degraded rapidly after it entered the cells. How could the effect continue after the agent was gone? Even more puzzling was that the effect spread inexplicably from the injected cells to the rest of the organism.
Rothman and Mello discussed these findings during Rothman’s brief visit. Then Rothman collected his worms and returned home. “I didn’t give it a thought after that,” he says. “But Craig did. And that is how two years later he found out what it was.”
No one appreciates the power of idle speculation more than scientists do. The scientific method, after all, consists in part of men and women just talking about ideas. In Mello’s office at the University of Massachusetts, he displays a hand-hooked rug designed for him by his sister. One day this spring, he traced a pattern on the rug with his fingers. The pattern showed several empty chairs connected with flowing lines.
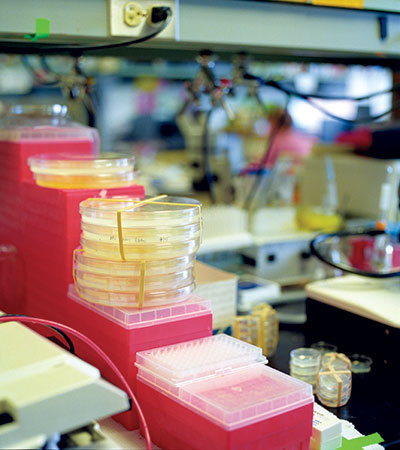
Sometimes, Mello likes to say, “really smart people are too smart. They know why an experiment won’t work before they try it. They’ve figured out that it would be impossible based on what they already know, not realizing that what they already know is flawed. You have to be ready to throw out everything you think you know. If you are comfortable, if you think you know something, then quite often if you get a weird result—like we did—you’ll discount it.”
Science, he adds, is always catching you by surprise: “This is the thing that is really humbling about using the scientific approach to learn about the world. You think you know how the world works, or how some biological mechanism works, but almost always you are totally wrong.”
Mello’s partner on this project was Stanford’s Andrew Fire, with whom he had earlier developed a microinjection technique for inserting DNA into C. elegans. Because Fire’s lab was California, the two men spoke on the phone for hours, day after day. “We had conversations so long,” Mello recalls, “my ear would be hurting and I’d be switching ears.”
One day, Fire suggested a possible explanation for what they were observing: what if the RNA they were injecting wasn’t pure? Perhaps the anti-sense RNA was contaminated with sense RNA, or vice versa. This would cause the molecules of single-stranded sense and anti-sense in the same solution to bind together chemically and form double-stranded RNA (dsRNA) molecules. Perhaps dsRNA was the real trigger of the potent gene silencing. It was a bold assertion, and Mello admits that when he first heard it, “I was actually more against the idea than I was for it.”
They put the idea to the test. They targeted mRNA that encodes a protein involved in C. elegans’s mobility. They injected purified sense RNA into some worms, and purified anti-sense into others. Neither affected the worms or their offspring. But when they injected sense and anti-sense simultaneously, the offspring of the worms twitched, indicating the team had successfully blocked the muscle-protein gene.
Fire had been right. The dsRNA was the trigger. And it turned out to be remarkably effective. As Fire and Mello narrowed their research, they discovered that just a few molecules of dsRNA could shut down almost any gene in the worm. “The key thing we found was that there was an active response in the organism,” said Mello. “It was doing something to turn the genes off.” Fire and Mello called that something RNA interference, or RNAi. They published their results in Nature in 1998.
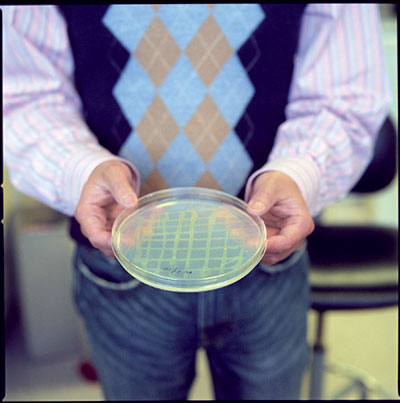
To better understand what was going on, Mello and Fire turned their attention to locating the genes responsible for RNAi. The C. elegans genome has about 20,000 genes. To find out which ones governed RNAi, Mello’s lab relied on a technique from classical genetics: introduce random mutations into a statistically large enough population and then look for mutants missing the characteristic associated with the gene you’re hunting. By examining the DNA of those mutants, you should be able to detect which gene was changed.
Mello’s lab exposed worms to a chemical that causes nucleotide changes in the DNA. “To hit every gene and every nucleotide in the whole worm genome, you have to apply the chemical to literally millions of worms,” Mello explains. Once they had produced a population of mutant worms, they fed them bacteria containing dsRNA designed to target a gene required for the development of embryos. As expected, most of the embryos died. But those that lived had a genetic difference: a mutation in an RNAi gene that protected them from the dsRNA. The lab then cloned and identified the gene, which turned out to be one active in many types of organisms, including humans.
Mello and Fire had discovered the key to a security system known as RNA interference, which intercepts and eliminates RNA with potentially dangerous genetic codes. Within months of the pair’s discovery, researchers working separately on fungi and flies confirmed that triggers other than dsRNA was setting off RNA interference in their specimens as well. This discovery—that their research applied across various classes of living things, from roundworms to people—was, Mello says, “the Eureka! moment.”
Professor of Biology Susan Gerbi explains that before RNAi was discovered, there was no effective one-size-fits-all way of switching off genes. For popular, well-researched organisms, scientists might have lots of methods to choose from, but for unpopular organisms there might be just a few. “[Switching off genes] was quite laborious, tedious, and time consuming,” Gerbi says. “The advantage of RNAi is that it can shortcut all of that, and it’s much more widely applicable.”
Today you can order dsRNA online. If you know the sequence of a gene (the entire human genome had been mapped by 2003), you can order its corresponding synthetic dsRNA, deliver it to the cells, and—presto—the gene is silenced. RNAi liberated genetic research.
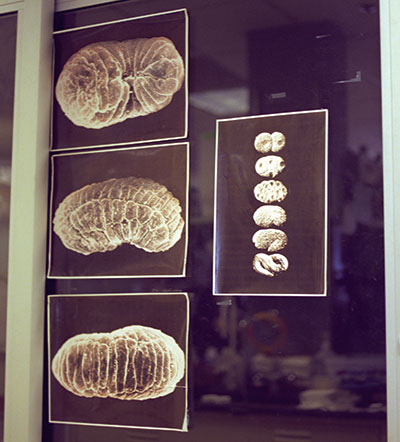
When the call came from the Nobel committee, it was well before dawn in Massachusetts. Mello happened to be awake, checking on his six-year-old daughter, Victoria, who is diabetic and requires blood sugar monitoring during the night. His wife, Edit, answered the phone. Thinking it was a crank phone call, she immediately hung up. But Mello knew the prizes were being announced and was quick to answer when the committee called back.
He described his reaction to the phone call when he spoke to the Shrewsbury High School students: “Total disbelief.” He admitted the award didn’t come as a complete surprise. “It had been talked about a lot,” he said. But he didn’t expect it so soon. “I thought maybe I’d be seventy.” Fire and Mello received the Nobel Prize just eight years after publishing their paper, even faster that Watson and Crick received it for discovering DNA.
“I can tell you one thing,” Frank Rothman says. “It is wonderful for a professor if one of his students wins a Nobel Prize. I was just absolutely thrilled.”
Mello’s decision to attend Brown in the first place was a natural one. His parents, James F. Mello ’58 and Sally A. Cameron-Mello ’58, met on campus, and his two older siblings are Jeanne M. Day ’80 and Frank J. Mello ’81. The family dinner table in Fairfax, Virginia, often resembled a Brown seminar. Mello’s father was a paleontologist—he worked at the Smithsonian—so the conversation often turned to science. “The kids all had opinions,” Sally says. “Craig and Jim did an awful lot of the arguing about science or issues. Craig kept us all very honest. He wouldn’t let you get away with saying something unless you had proof for it.”
When it was his turn to attend Brown, Craig Mello met many teachers who inspired him, including Rothman, Gerbi, and Professor of Biology Kenneth Miller. Mello majored in biochemistry, but says it was molecular biology that in the end captured his imagination. “It was DNA, and the power of the genetics of DNA, and what you could do with it that really hooked me on biology.”
Since winning the prize, Mello has had trouble sleeping. “My brain races,” he said. “It came with a real sense of responsibility.” Mello looks at the world and sees a fragile planet and an unstable society badly out of equilibrium with its environment. “We can’t sustain what we’re doing,” he says. “Our survival is on the line.” From the overharvesting of oceans to species extinction and global warming, there is a lot to keep Mello awake. “We could go from functioning beautifully, with an incredibly high standard of living, to a society that collapses devastatingly—imagine Western culture like ours with actual starvation and famine. It could happen.”
Mello believes that science can lead the way out of this mess, but it needs adequate funding. In his effort to persuade the government of the importance of properly supporting scientific research, Mello has even met with Vice President Dick Cheney—and he has a picture to prove it. Mello asked Cheney to provide him with a copy, he says, because otherwise no one who knows him would believe the meeting took place. “As far as my politics, I’m about as Democratic as you’ll ever see,” Mello says. “But I will talk to anybody about getting more funding for science because it is so important.”
His dream is to get more people talking, and then get them to talk some more. Put climate or energy scientists into a room with economists, policy makers, and social and political scientists, he suggests. Society’s problems, he insists, are “a social engineering problem. How do you get societies to function for the common good instead of self-interest? It’s not easy. To incubate these kinds of ideas, you really need an academic environment.”
And in science, he insists, even complete certainty can be elusive. But it’s not a reason for delay. “There will always be somebody who says global warming isn’t happening,” he says, “who will make an academic career as the guy they always invite when they want somebody to say it isn’t happening.” Disagreements are healthy, he says, but at the same time institutions could be solving the problems that people do agree on. “Brown, with its outstanding geological sciences program, should be a leader in putting together this kind of program.”
Another thing that changed after Mello won the Nobel Prize was his phone number. Suddenly he was spending all day answering the phone. Calls came from all over the world, many of them from people who with genetic diseases or a close relative dying of cancer.
“It does hit you,” he says, his voice faltering. “You realize how many people are hurting because of diseases that we could do something about, that we can cure. It really makes you want to get right back to work.
Linda Heuman is a BAM contributing editor.